Small modular reactors (SMRs) represent a promising advancement in the pursuit of safer, more efficient nuclear power. These compact reactors leverage natural circulation for passive cooling, reducing the reliance on active systems like pumps. This design philosophy is widely considered to enhance SMR safety by mitigating the risk of overheating. Unlike traditional large reactors, SMRs can be easily deployed in diverse regions, providing cost-effective and flexible energy solutions for areas with lower energy demands. The IAEA defines SMRs as reactors with up to 300 MWe output, and their smaller size allows for easier installation, quicker construction, and improved safety features. Natural circulation reactors utilize buoyancy-driven flow to remove heat without the need for external power, reducing the likelihood of failures. However, there are still challenges in modeling and testing the reliability of these passive systems, particularly concerning their potential flow instabilities at lower pressures. Ongoing research in projects like ANItA seeks to improve modeling accuracy and assess the viability of SMRs for predictive safety analysis. As the demand for carbon-neutral energy grows, SMRs offer a sustainable solution that could pave the way for the future of nuclear power.
Proof is necessary, but the quiet design philosophy used in small compact reactors can lead to a safer, more affordable future for nuclear power.
The foundation of contemporary society is energy production. Many of the world has a higher quality of life today than it has ever been at any additional time in human history thanks to the support of industries and technologies that have considerably increased worker productivity. The cost, however, will be rough: The vast majority of energy has been produced by burning various fossil fuels such as coal, oil, and natural gas, releasing huge quantities of carbon dioxide into the atmosphere, as well as a plethora of harmful and asthma-inducing substances. Despite having a strong impact on people’s health, carbon dioxide still functions as a greenhouse gas trapping thermal radiation energy and warming the atmosphere.
The majority of life on our planet is threatened by this process, which is sometimes referred to as global warming, including both ourselves and our way of life. The medical community has almost unanimously agreed that carbon dioxide serves as a greenhouse gas, that mortal industrial activity has caused global warming, and that global warming has a general negative impact on life on Earth.
Little modular reactors: A modern solution?
Some nations are quickly researching and implementing carbon-neutral energy policies in an effort to reduce further harm to the environment and with a view to improving energy security. This has led to a renewed interest in nuclear technologies, manifesting in research spanning the entire spectrum of the field, from superior Generation-IV reactor designs to accident-tolerant fuels and closed-fuel cycles.
A common thread of this resurgence, yet, is a trend towards smaller designs, which, it is hoped, will overcome the difficulties faced by the nuclear industry in commissioning fresh plants. In order to expand the knowledge about the safety of these smaller designs, known as small modular reactors ( SMRs ), Project C1 within ANItA, a Swedish national competency center for nuclear power technology.
SMRs, which the IAEA widely refers to as reactors with power capacities up to 300 MWe and can be factory-fabricated and transported as modules, have a number of advantages over their larger counterparts.
Second, big reactors are not a realistic option for many localities, by reducing the size and cost, SMRs provide flexibility, allowing for the introduction of nuclear power to regions that do not need the huge output of large reactors. Next, SMRs usually enjoy much streamlined designs thanks to their smaller, more easily cooled cores, this, combined with their factory fabrication, can reduce construction times.
Lastly, by reducing interest payments and time without revenue, these factors combined can help offset the diminished economies of scales created by using a smaller design. The region of interest and the ability of the manufacturers to scale production will probably determine whether these factors will make SMRs more competitive with large reactors.
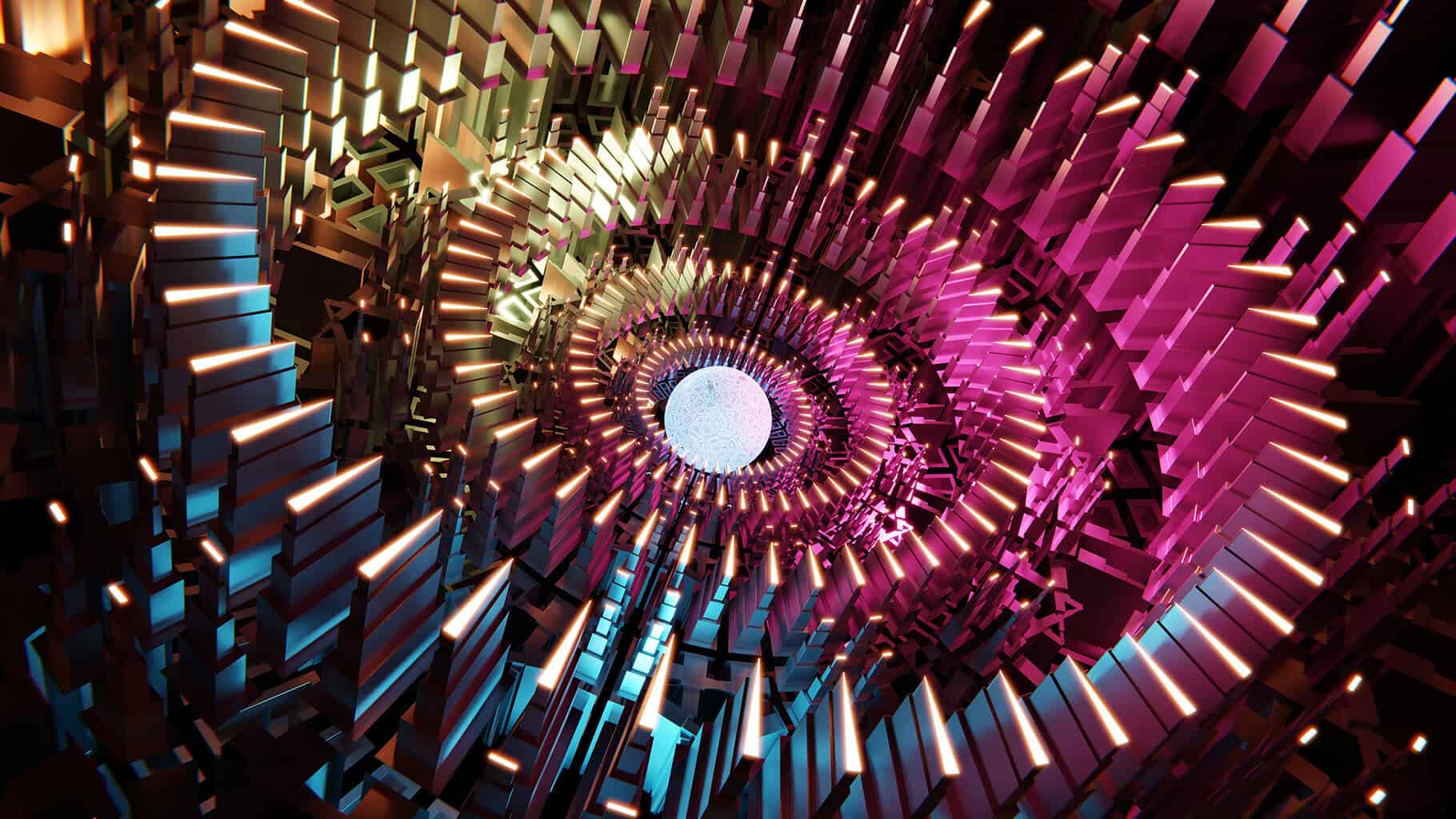
But, ANItA Project C1’s main concern is that because their smaller energy output allows for passive cooling of the shutdown core totally, which rely on healthy circulation. This contrasts favorably with Generation III and older plants, which rely on active systems ( such as pumps ) to remove decay heat from the core after the reactor is shut down.
While some large Generation designs ( such as the AP1000 and ESBWR ) use passive cooling systems, passive systems are practically standard in light water SMR concepts.
This design philosophy is generally believed to increase safety, 4 reducing the risk of a serious accident, and 4 allowing for plant modifications that might improve the economic viability of SMRs. For the purposes of district heating and desalination, this includes co-sitting several reactors close to one another or locating plants closer to population centers.
However, this will necessitate the adoption of new laws and licensing procedures for SMRs, which will require the use of rigorous scientific evidence to support the claims of superior safety. Evaluating for evidence is one goal of ANItA, with research in this project being conducted at the Royal Institute of Technology in Stockholm, in collaboration with Vattenfall, Westinghouse, and the rest of the ANItA competency centre. On this basis, it is appropriate to bring up silent systems and healthy circulation.
Quietly safe through organic circulation
The IAEA defines a silent system as either a system made entirely of quiet components and structures or a system that only uses active components to initiate passive operation in its place. 4 While philosophical SMR designs use a variety of passive elements to improve safety, like as accident-tolerant fuels, nearly all gentle water SMR designs use organic circulation in some way as a silent mechanism to achieve their safety objectives.
Passive systems are generally regarded as more reliable than their active counterparts because they don’t rely on an active power source. However, the methods with which the reliability of a system is most often evaluated, e. g., using fault tree analysis with historical data for failure rates of energetic system components, can be difficult to apply to silent systems.
First, there is no such set of historical data to use to determine the failure rate of some quiet functions. Next, as a silent mechanism may operate between what is typically characterized as success or failure, the definition of passive system failure may not be as properly defined. Thirdly, and lastly, is it still a question whether or not current reactor system codes can be used to model silent systems for safety analysis and licensing purposes.
These ideas are immediately applicable when compared to the normal circulation-driven cooling systems used to power many SMR designs, which operate on the basis of buoyancy-driven flow. In general, hotter water and steam are less dense and will typically flow up, whereas colder water is more dense and will typically flow down. As a result, by placing the heat sink (e.g., a heat exchanger that will transfer heat to the atmosphere ) above the heat source (such as the reactor core or another heat exchanger if the system is designed to cool the containment), we can ensure flow from the latter to the former, accomplishing the function of a pump without an external source of power.
So, natural circulation does not have a traditional “failure rate” even though there are component failure rates for components of the system that are essential for establishing system flow, such as valves that need to be opened or heat exchangers that cannot be blocked.
Also, even if enough flow is not established during a casualty to prevent damage to the plant, some limited flow may still exist, which can slow down accident progression, give more time for accident management, and limit damage to the plant. Lastly, while system codes can simulate natural circulation, the results are generally less accurate than those of forced circulation systems models.
While these concerns are themselves legitimate, one may yet ask,’ Why does this matter? If organic circulation guarantees flow, why bother with a failure rate, and what difference do minor modeling errors make? A possible concern regarding normal circulation is its susceptibility to flow instabilities, particularly at lower system pressures, effectively, the flow rate can oscillate, or even reverse, in a chaotic fashion.
Additionally, these oscillations may result from variations in a large number of system parameters, some of which will vary depending on the need for the affiliated system. For their common adoption, it will be needed to assess the impact these oscillations may have on the safety function of a healthy circulation system.
Ongoing research
A varied approach will be necessary to resolve these intersecting issues. To summarise the research objectives of ANItA project C1:
Justify system codes
We can improve the models and assess uncertainties of predicted healthy circulation flow rates and heat removal efficiency by simulating thermal-hydraulic experiments and tests carried out on administrative nuclear power plants using linear codes for safety analysis in order to achieve satisfactory safety margins in SMR designs that rely on healthy circulation.
Assess the dependability of biological circulation systems With the assurance that our codes will be reliable, we can model safety systems driven by healthy circulation and assess their dependability. This can be accomplished by using a variety of techniques, including running several simulations under different system parameters with different uncertainty levels, such as confidence intervals in relation to material property measurements. We can calculate the likelihood of a system failure while also evaluating the design’s robustness using quantitative methods and stochastic safety codes.
Analyze a generalized SMR of boiling water
It would be fascinating to evaluate an entire SMR design in order to compare their safety to that of their larger counterparts, in addition to modeling a silent safety system. A boiling water reactor would be most intriguing in this situation because more recent designs, like the ESBWR and BWRX-300, both by GE Hitachi, use healthy circulation to propel flow through the core during regular operation. Such systems would have both scientific and business value if they were tested for their stability and load-following characteristics as well as a full safety analysis.
Compiling the results of this research will provide designers, regulators, policymakers, and researchers in adjacent fields with the necessary context to form an educated opinion on the reliability of healthy circulation-based quiet safety systems. It is hoped that this work will lay the groundwork for enhancing sunshine water reactor safety and facilitating the introduction of new nuclear power in Sweden in an effective and timely manner.